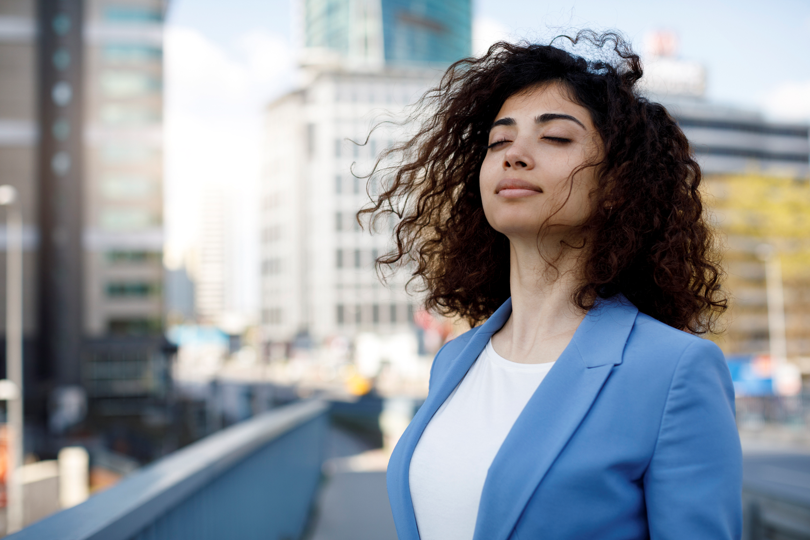
What do air purification and disinfection systems do?
Air purification and disinfection systems are being designed to effectively clean the air in the environment and reduce or inactivate harmful populations of airborne pathogenic microorganisms, aiming to provide significant assistance in the fight against outbreaks and harmful infections.
The objective of these systems is to improve infection prevention and control measures that the air disinfection systems aspiring to play comes with an understandably high expectation for reliable and reproducible efficacy data. All this is required for when you are faced with a seamless technology implementation plan, together with robust efficacy data, you would feel highly confident that ‘You’re off to a good start!‘ And whilst identifying and overcoming pain points for successful technology implementation is challenging,
I see that the latter is a lower barrier to entry than the ability to obtain and provide reliable efficacy data. Understandably, the questions that infection prevention professionals are raising are focused on ‘How can we ensure that efficacy has been achieved and how do we know that airborne pathogens have been eradicated from the area?‘ We’ve listened to feedback from healthcare professionals and read through reports and findings from the scientific community to determine how reliable are the claims around air disinfection systems efficacy.
Transmission of microorganisms by air
Bioaerosols are a loosely defined group of airborne particles of biological origin, generally including bacteria, fungi, and viruses, as well as pollen, their fragments, and various antigens. They can cause many adverse health effects, including allergic, toxic, and infection responses.
Exposure to bioaerosols may be especially hazardous in clinics and hospitals. In addition to SARS-CoV2 and influenzas virus, some bacteria such as Streptococcus pyogenes, Neisseria meningitidis, Corynebacterium diphtheria, and Mycobacterium tuberculosis are known to be transmitted predominantly by airborne droplets from infected people, and they may cause nosocomial infections such as surgical site infections, and respiratory and urinary tract infections.
Under the influence of controllable and uncontrollable factors, indoor air quality is in a constant state of flux. The individual human microbiome, ventilation, outdoor air, plumbing systems, and foliage all contribute to the airborne particulate burden. For example, tuberculosis is transmitted person-to-person by the airborne route via small particle aerosols measuring 1 to 5 μm, sufficiently small that they remain aloft in the air.
International literature data report that the increase of infectious risk may be due to heating, ventilation, and air conditioning (HVAC) systems contaminated by airborne pathogens. Contamination could occur at the duct, ventilation grille, and filter levels as a result of dust, oil, water and resin accumulation.
Air filtration and purification standards
There are several standards for optimum air filtration and purification published by the International Organisation for Standardisation (ISO), outlining unacceptable ranges of particulate sizes. According to ISO8573-1:2010, in each cubic metre of compressed air, the particulate count should not exceed 20,000 particles in the 0.1 – 0.5 micron size range, 400 particles in the 0.5 – 1 micron size range and 10 particles in the 1 – 5 micron size range.
However, there seems to be less attention focused on air disinfection systems and how to test for microbial efficacy of a certain air disinfection unit. In other words, how to ensure that air disinfection has been achieved. It has been noted that a colony forming unit (CFU) count that exceeds the threshold, prompts an evaluation of cleaning practices, personnel work practices, operational procedures, and air-filtration effectiveness.
In the direct compounding area (ISO 5 classification), 1 CFU/m³ bacteria or fungus is considered actionable. In the surrounding anteroom (ISO 7 classification), the threshold is higher at >10 CFU. Although, it wasn’t exactly outlined how to test for the colony forming units and how to ensure these values are kept to a minimum. Therefore, I’ll aim to shed light on the different testing methods for the evaluation of microbial count in HVAC systems and air purification units.
Vacuum pump test method
Sampling of bioaerosols can be performed by impaction onto growth media, impingement into liquid, or capture by filtration. The most common samplers in each category are used at flow rates between 2 and 22 L min¹ using a vacuum pump or personal pump.
While impaction and impingement devices are more commonly used for bioaerosol sampling due to their ability to preserve microbial viability and often serve as reference samplers in evaluating other sampling devices.
For instance, in one study by Holopainen and colleagues (2001), the amount of dust in category P1 and P2 ducts from different sampling sites was measured by using the vacuum pump test method. They found that the ducts without residual oil had lower amounts of accumulated dust, indicating that the demand for oil free components in the cleanliness classification is reasonable.
Static chamber test method
Alternatively, one of the main methods reported in the literature is the static chamber test method developed by Foarde et al. (1994), to quantitatively evaluate the growth of microorganisms on HVAC test materials at controlled RH and temperature.
One study by Chang et al.(1997) evaluated the susceptibility of three types of ventilation duct materials (fibrous glass ductboard, galvanised steel, and insulated flexible duct) to fungal (P. chrysogenum) growth in the presence of soiling, dust and high relative humidity, using the static chamber test. Each sample was inoculated with spores of P. chrysogenum and incubated in a static chamber (32 x 39 x 51 cm) controlled at 97% relative humidity (RH) and 21°C for six weeks. T
he conditioned test materials were either directly inoculated with approximately 1 x 10⁵ CFUs of P. chrysogenum per sample block or treated (e.g., by wetting or soiling) and then inoculated. Sterilised but uninoculated blocks were used as controls. Saturated salt solutions were used to maintain specific RHs in each chamber. To quantify the fungal growth, triplicate inoculated and duplicate uninoculated blocks were removed from each chamber for analysis on days 0, 1, 7, 14, 21, 27, 35, and 42.
They found that after artificial soiling with the equivalent of approximately 0.4 to 0.7 mg cm² HVAC dust, fungal growth as measured by an increase of culturable spores of more than one order-of-magnitude was detected on the flexible duct and the fibrous glass duct board but not the galvanised steel.
On galvanised steel samples soiled with significantly more dust, approximately 9-18 mg cm², moderate growth (one order-of-magnitude increase in culturable spores) was seen. Conditions favourable for fungal growth in HVAC ducts exist because, with current HVAC design and operation, dust penetration into and accumulation on the duct surface is almost inevitable. Humid conditions can be found around cooling coils, humidification zones, and sometimes outside air intakes when the outdoor air is humid or foggy.
HVAC testing facility
Correspondingly, Farnsworth and colleagues (2006) evaluated the effectiveness of building air handling units (AHUs) in serving as high volume sampling devices for airborne bacteria and viruses.
An HVAC test facility constructed according to ASHRAE Standard 52.2-1999 was used for the controlled loading of HVAC filter media with aerosolised bacteria and virus. Non-pathogenic Bacillus subtilis var. niger was chosen as a surrogate for Bacillus anthracis. Three animal viruses; transmissible gastroenteritis virus (TGEV), avian pneumovirus (APV), and fowlpox virus were chosen as surrogates for three human viruses; SARS coronavirus, respiratory syncytial virus, and smallpox virus respectively.
These bacteria and viruses were nebulised in separate tests and injected into the test duct of the test facility upstream of a MERV 14 filter. SKC Biosamplers upstream and downstream of the test filter served as reference samplers.
Their results indicate that the airborne concentration of spore-forming bacteria in building AHUs may be determined by analysing the material collected on HVAC filter media, however culture-based analytical techniques are impractical for virus recovery. Therefore, molecular-based techniques such as the polymerase chain reaction (PCR) technique could be used to identify virus nucleic acid on HVAC filter media.
Bioaerosol samplers
One study in a large tertiary-care hospital provided insights into aspergillus surveillance of contaminated HVAC systems in different hospitals compartments using bio samplers and HEPA filter vacuum dust bags. They found that the higher concentrations of aspergillus seen inside the hospital compared with outdoors and the various moisture/HEPA filter/renovation incidents suggest that numerous small to moderate sources of aspergillus exist in the hospital.
A total of 842 bacterial and fungal samples were collected using one stage bioaerosol sampler. For each air sample, 0.050 m³ to 0.400 m³ of air was collected, depending upon the expected fungal concentrations. Samplers were loaded with autoclaved malt extract agar medium for fungal isolation and incubated at 25°C for three days, after which time the number of viable fungal colonies were recorded and then analysed using light microscope. Aspergillus spp., Penicillium spp. and several other fungal genera were classified to species with the help of standard references.
Moreover, seventy-four dust samples were collected from portable HEPA filter vacuum bags used by hospital maintenance staff to clean the duct covers in patient rooms and other areas. Approximately 100 mg of dust was taken out of each bag and analysed using the sandwich ELISA method of Arruda.
This method measures the asp f1 protein, which is found in the mycelia of A. fumigatus but not in the spores. Authors found that highest indoor concentrations of mean total indoor fungi (192.8 cfu/m³) and A. fumigatus (11.0 cfu/m³) were collected in the rooms that were undergoing duct cleaning. This suggests that the ducts are a significant source of A. fumigatus spores and fungal spores in general.
Correspondingly, studies that evaluated the use of air disinfection units in HVAC systems analysed the efficacy of these systems using Bio-samplers. A study that employed an innovative air purification technology that uses a shielded UV-C light lamp to continuously purify the air, reported significant decrease for bacterial and fungal particles.
The researchers evaluated microbial efficacy by using Surface Air System microbial air samplers to influence 1,000 L of air onto agar plates and were refrigerated and shipped to an accredited independent laboratory where they were incubated for 5 days at 32°C ± 2°C.
All bacteria and fungi CFUs were tabulated by the laboratory using published guidelines. In addition, Cheng and colleagues (2020) examined the efficacy of three air disinfections methods using ultraviolet germicidal irradiation (group A), plasma circulation air steriliser (group B), and ultraviolet germicidal irradiation plus plasma circulation air steriliser (group C). All three methods had significant disinfection effects, with group B showing the best disinfection effect for the longest duration in sterilizing the air in a CT machine room.
Efficacy results were tested by placing nutrient agar plates inside a microbial air sampler to collect air samples from the tested room. After sampling, the nutrient agar plates were placed in a 37°C incubator for 48 h; then, the number of colonies and total number of bacteria were counted.
Final thoughts
Air disinfection and purification systems vary and can be combined to deliver better outcomes. However, accumulation of dust and dirt in HVAC units possess a dangerous risk of infection of patients in healthcare settings. Many interventions have been proposed in the literature and reported significant decrease in rates of infections when combined with HEPA filters in HVAC units, especially the use of in-duct UV-C lamps. However, the main challenge for these disinfection systems is how to test and ensure that disinfection has been achieved.
As we’ve highlighted, tests are being continued to evaluate claims around air disinfection systems efficacy, a commendable activity particularly important as we look to combat the transmission of COVID-19. We look forward to seeing this encouraging line of research continue and hope to soon see the development of industry standards to help regulate statements of infection and prevention control.